Transgenic Approaches for Bacterial Resistance
In the ongoing battle against plant pathogens, scientists have turned to genetic engineering to develop crops that can resist bacterial infections. These transgenic approaches for bacterial resistance offer innovative solutions to protect plants from devastating diseases, ensuring food security and agricultural sustainability.
This blog post delves into various strategies employed to confer bacterial resistance in plants, focusing on RNA interference (RNAi), detoxification, antimicrobial peptides, cell wall modification, oxidative bursts, ATP/ADP transporters, and pattern recognition receptors. By exploring these transgenic approaches for bacterial resistance, we can better understand how modern science is equipping plants to fight off harmful bacterial pathogens.
1. RNA Interference (RNAi): A Revolutionary Tool for Plant Protection
Mechanism
RNA interference (RNAi) is a groundbreaking genetic tool that allows scientists to silence specific genes within pathogens, effectively disrupting their ability to cause disease. This process involves introducing small interfering RNA (siRNA) or short hairpin RNA (shRNA) into plants.
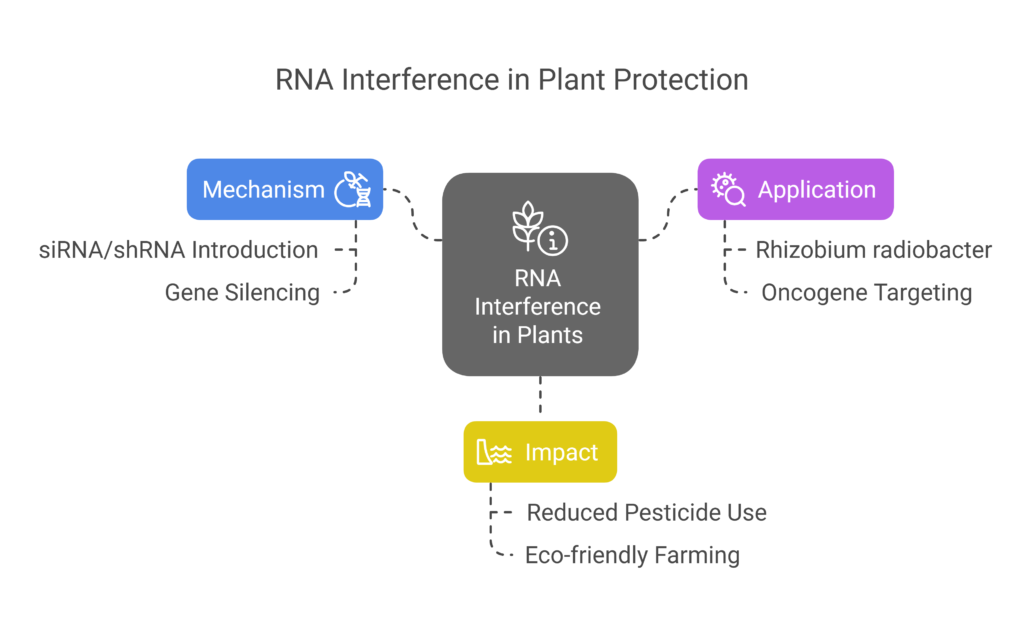
These RNA molecules are designed to be complementary to the mRNA of bacterial genes that are crucial for virulence. Once inside the plant cells, the RNAi machinery recognizes these complementary sequences and degrades the targeted mRNA, preventing the expression of harmful bacterial genes. This targeted gene silencing can significantly reduce the pathogen’s ability to infect and damage the plant.
Example: Targeting Rhizobium radiobacter (formerly Agrobacterium tumefaciens)
One of the most notable applications of RNAi in plant protection is its use against Rhizobium radiobacter, the bacterium responsible for crown gall disease. This pathogen transfers oncogenes, such as iaaM and ipt, into the plant genome. These oncogenes encode enzymes that disrupt the plant’s hormone balance, leading to uncontrolled cell division and the formation of tumors, or galls, on the plant.
By using RNAi to silence these oncogenes, scientists can prevent the hormone imbalance and subsequent tumor formation. Transgenic plants engineered to express siRNA or shRNA targeting iaaM and ipt effectively inhibit the expression of these genes, thereby conferring resistance to crown gall disease. This RNAi-mediated silencing not only stops tumorigenesis but also helps maintain the plant’s normal physiological functions, ensuring healthier growth and development.
Impact and Potential
The potential of RNA interference in plant protection is demonstrated by its application in the fight against Rhizobium radiobacter. RNA interference (RNAi) provides an accurate and efficient way to manage bacterial infections by selectively targeting and inhibiting virulence genes. By lowering the dependence on chemical pesticides and minimizing the possibility of off-target impacts, this strategy encourages more environmentally friendly farming methods.
2. Detoxification: Engineering Plants to Neutralize Bacterial Toxins
Mechanism
One of the key strategies in transgenic approaches for bacterial resistance is detoxification. This involves genetically modifying plants to produce enzymes that can neutralize or break down harmful toxins secreted by bacterial pathogens. By expressing these detoxifying enzymes, transgenic plants can effectively counteract the damaging effects of bacterial toxins, thereby enhancing their resistance to infections.
Examples
a). Phaseolotoxin
The pathogen Pseudomonas syringae pv. phaseolicola produces phaseolotoxin, a potent toxin that inhibits the enzyme ornithine carbamoyltransferase, disrupting the urea cycle in plants and causing chlorosis and stunted growth. To combat this, transgenic tobacco plants have been engineered to express a bacterial gene encoding phaseolotoxin-resistant ornithyl transcarbamoylase. This enzyme remains functional in the presence of phaseolotoxin and effectively degrades the toxin, safeguarding the plant from its detrimental effects and enhancing resistance to infection.
b). Tabtoxin
The pathogen Pseudomonas syringae pv. tabaci produces tabtoxin, a toxin that, upon hydrolysis, releases tabtoxinine-β-lactam, which inhibits glutamine synthetase. This inhibition disrupts nitrogen metabolism, resulting in chlorosis and tissue damage. To counteract this, transgenic plants can be engineered to express detoxification genes that neutralize tabtoxin. These genes encode enzymes capable of modifying or degrading the toxin, thereby preventing it from inhibiting glutamine synthetase and protecting the plant from the harmful effects of the infection.
c). Albicidin
The pathogen Xanthomonas albilineans produces albicidin, a toxin that inhibits DNA gyrase, an enzyme crucial for DNA replication. This inhibition disrupts cell division and plant growth, leading to leaf scald disease. To combat this, enzymes capable of breaking down albicidin have been introduced into transgenic plants. These enzymes degrade the toxin, preventing it from inhibiting DNA gyrase and thereby protecting the plant from infection and its damaging effects.
Impact and Potential
The detoxification strategy exemplifies the power of genetic engineering in enhancing plant resistance to bacterial pathogens. By equipping plants with the ability to neutralize harmful toxins, scientists can significantly reduce the impact of bacterial infections on crop health and yield. This approach not only provides a direct method of combating bacterial toxins but also reduces the need for chemical treatments, promoting more sustainable agricultural practices.
3. Antimicrobial Peptides (AMPs)
Mechanism
Antimicrobial peptides (AMPs) are small, naturally occurring proteins that play a crucial role in the innate immune system of plants. These peptides directly kill or inhibit the growth of a wide range of pathogens, including bacteria, fungi, and viruses.
AMPs achieve this by disrupting the pathogen’s cell membrane, leading to cell lysis, or by interfering with essential intracellular processes. When expressed in transgenic plants, AMPs provide broad-spectrum resistance, offering a versatile defense mechanism against multiple types of pathogens.
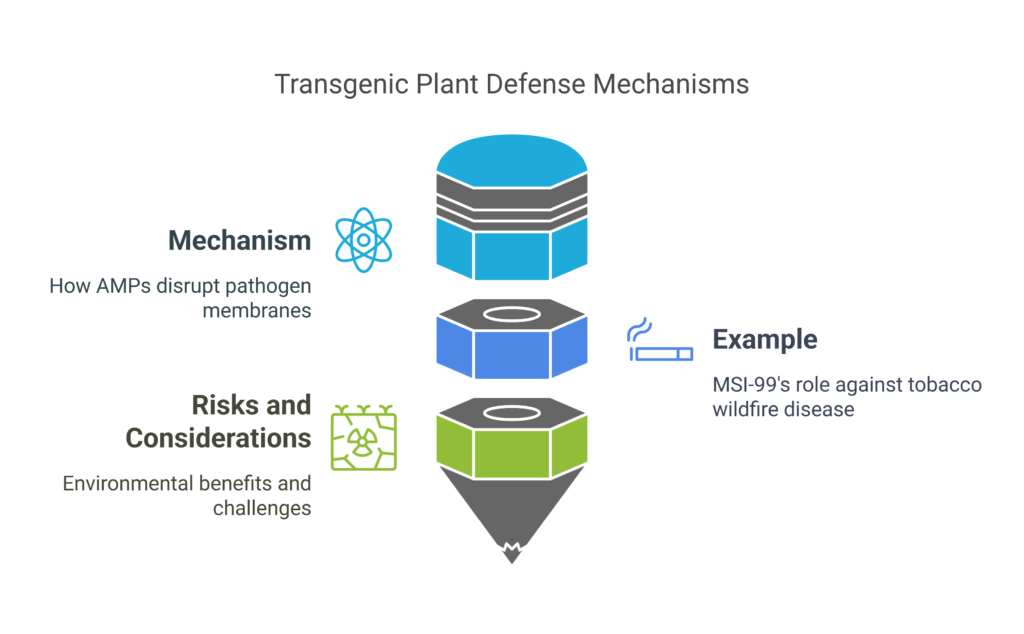
Example:
One notable example is MSI-99, a synthetic antimicrobial peptide derived from magainin 2, which is found in the skin of the African clawed frog. MSI-99 has been expressed in transgenic plants to combat Pseudomonas syringae pv. tabaci, the causative agent of wildfire disease in tobacco. The peptide disrupts the bacterial cell membrane, effectively killing the pathogen and protecting the plant from infection.
Risks and Considerations:
The potential of RNA interference in plant protection is demonstrated by its application in the fight against Rhizobium radiobacter. RNA interference (RNAi) provides an accurate and efficient way to manage bacterial infections by selectively targeting and inhibiting virulence genes. By lowering the dependence on chemical pesticides and minimizing the possibility of off-target impacts, this strategy encourages more environmentally friendly farming methods.
4. Cell Wall Modification
Mechanism
Another effective strategy for enhancing plant resistance to bacterial pathogens is modifying the plant cell wall. The cell wall serves as the first line of defense against invading pathogens. By strengthening the cell wall, plants can resist bacterial penetration and degradation, making it more difficult for pathogens to establish an infection.
Examples
a). Peroxidase
Peroxidase enzymes are crucial for strengthening the plant cell wall by catalyzing the cross-linking of components like lignin and suberin, making the cell wall more resistant to mechanical penetration and enzymatic degradation by pathogens. Transgenic plants engineered to express high levels of peroxidase have demonstrated increased resistance to pathogens such as Erwinia carotovora, the causative agent of soft rot disease. The reinforced cell wall integrity effectively prevents bacterial penetration, protecting the plant tissues from infection and damage.
b). Polygalacturonase Inhibitors
Polygalacturonase inhibitors (PGIPs) function by targeting polygalacturonase, an enzyme produced by pathogens like Pseudomonas syringae pv. tabaci that degrades pectin, a key component of the plant cell wall. By inhibiting this enzyme, PGIPs prevent the breakdown of the cell wall, maintaining its structural integrity. Transgenic plants engineered to express PGIPs can effectively counteract the action of polygalacturonase, enhancing resistance to Pseudomonas syringae pv. tabaci and protecting the plant from the damaging effects of the pathogen.
Impact and Potential
Both AMPs and cell wall modification represent powerful tools in the genetic engineering arsenal for enhancing plant resistance to bacterial pathogens. AMPs offer broad-spectrum protection by directly targeting and killing pathogens, while cell wall modification provides a physical barrier that hinders bacterial invasion and degradation. Together, these strategies contribute to the development of more resilient crops, capable of withstanding bacterial infections and reducing the reliance on chemical pesticides.
5. Oxidative Burst
Mechanism:
The oxidative burst is a critical component of the plant’s innate immune response. It involves the rapid production of reactive oxygen species (ROS), such as superoxide anions (O₂⁻), hydrogen peroxide (H₂O₂), and hydroxyl radicals (OH•), at the site of pathogen invasion. These ROS molecules are highly reactive and can cause oxidative damage to the invading bacteria, leading to their death. The oxidative burst serves as an immediate and potent defense mechanism, effectively halting the spread of pathogens before they can establish a full-blown infection.
Key Processes
a). ROS Production
The oxidative burst is typically triggered by the recognition of pathogen-associated molecular patterns (PAMPs) by plant pattern recognition receptors (PRRs). This recognition activates enzymes like NADPH oxidases and peroxidases, which generate ROS.
b). Pathogen Killing
The high reactivity of ROS damages bacterial cell membranes, proteins, and DNA, leading to cell death. This rapid response can neutralize pathogens before they can cause significant harm to the plant.
Example: Engineering Plants to Enhance Oxidative Burst Against Erwinia carotovora
Erwinia carotovora is a pathogen responsible for soft rot disease in various crops, causing significant agricultural losses. To combat this, scientists have engineered plants to enhance their oxidative burst response. By overexpressing genes involved in ROS production, such as NADPH oxidases, transgenic plants can generate a more robust and rapid oxidative burst upon infection.
Impact and Benefits
a). Enhanced Resistance
Transgenic plants with an enhanced oxidative burst response show increased resistance to Erwinia carotovora. The rapid production of ROS effectively kills the bacteria, preventing the onset of soft rot disease.
b). Broad-Spectrum Defense
The oxidative burst is not specific to a single pathogen, making it a versatile defense mechanism. Enhanced ROS production can provide protection against a wide range of bacterial pathogens.
c). Sustainable Agriculture
By boosting the plant’s natural defense mechanisms, the need for chemical pesticides can be reduced, promoting more sustainable agricultural practices.
6. ATP/ADP Transporters
Mechanism: In all living cells, including bacterial infections, adenosine triphosphate (ATP) and adenosine diphosphate (ADP) are essential molecules for energy transfer. Plants can reduce the amount of energy available to invasive bacteria and impede their ability to multiply and spread disease by altering the transit of ATP/ADP. Targeting the bacterial systems that depend on host-derived ATP/ADP to meet their energy requirements is the goal of this tactic.
Pattern Recognition Receptors
Mechanism: Introducing receptors that recognize pathogen-associated molecular patterns (PAMPs) to trigger immune responses.
Example: The XA21 receptor in rice provides resistance against Xanthomonas campestris pv. musacearum by recognizing bacterial molecules and initiating defense responses.
Infection Routes of Phytopathogenic Bacteria
Understanding how bacteria infect plants is crucial for developing effective resistance strategies. Common infection routes include:
- Through stomata
- Through wounds
- Through hydathodes
- Through nectarthodes (bacteria in nectar)
Symptoms of Rhizobium radiobacter Infection
Infection by Rhizobium radiobacter leads to the synthesis of Indole-3-acetic acid (IAA), disrupting normal plant hormone balance and causing tumor formation.
RNAi-Mediated Oncogene Silencing
RNAi technology has been successfully used to confer resistance to crown gall tumorigenesis. By silencing oncogenes in transgenic plants, researchers have prevented tumor formation upon infection with Agrobacterium tumefaciens. This approach has been demonstrated in transgenic tomatoes and A. thaliana roots, highlighting the potential of RNAi in plant protection.
Conclusion
Transgenic approaches for bacterial resistance in plants offer a multifaceted strategy to combat bacterial pathogens. By leveraging RNAi, detoxification, antimicrobial peptides, cell wall modification, oxidative bursts, ATP/ADP transporters, and pattern recognition receptors, scientists can develop crops with enhanced resistance to bacterial diseases. These innovations not only protect plants but also contribute to sustainable agriculture and food security. However, careful consideration of potential risks, such as the evolution of resistance to antimicrobial peptides, is essential to ensure the long-term effectiveness of these strategies.