Introduction
Fungal diseases are a major hazard to agriculture worldwide, affecting food security and resulting in large crop losses. Enhancing fungal resistance in plants has emerged as a key area of current agricultural research to address these issues. In order to defend themselves against fungal diseases, plants have developed a variety of passive and active defense mechanisms.
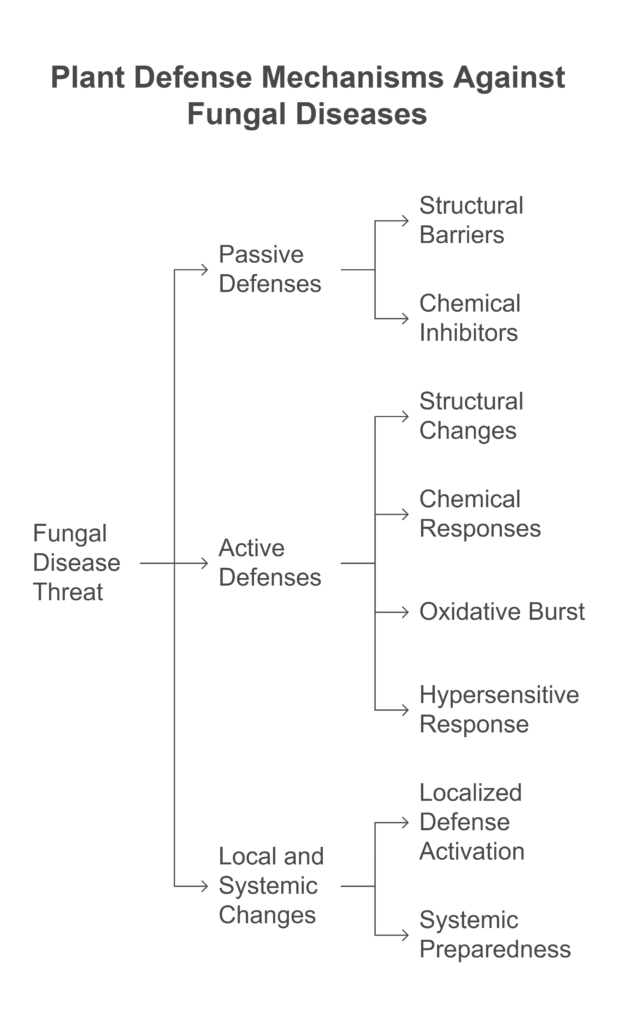
a). Passive Defenses
Passive Defenses are always present in plants and serve as the first line of defense against pathogens. These defenses include structural barriers like the cell wall, which physically block pathogen entry, and chemical inhibitors such as glucosides, saponins, and alkaloids, which deter or inhibit pathogen growth. These preformed mechanisms provide a constant level of protection, helping plants resist infections even before pathogens can establish themselves.
b). Active (Inducible) Defenses
Active Defenses are triggered in response to pathogen attacks and involve a range of mechanisms to combat infections.
These include structural changes such as the deposition of callose, suberin, and lignin in the cell wall to strengthen barriers, chemical responses like the production of phytoalexins, pathogenesis-related (PR) proteins, chitinase, and glucanase enzymes that directly target pathogens, an oxidative burst that rapidly generates reactive oxygen species to kill invading pathogens, and the hypersensitive response, which induces localized cell death to isolate and prevent the spread of the pathogen.
These inducible defenses provide a dynamic and targeted approach to protecting the plant from further damage.
c). Local and Systemic Changes
Local and Systemic Changes refer to the plant’s coordinated defense responses, which occur both at the site of infection (local) and across the entire plant (systemic) to enhance overall resistance. Locally, the plant may activate mechanisms like the hypersensitive response or strengthen cell walls to contain the pathogen, while systemically, it can trigger widespread changes such as the production of defense-related proteins or signaling molecules that prepare the entire plant for potential future attacks. This dual approach ensures a robust and comprehensive defense against pathogens.
Callose Deposition and Reactive Oxygen Species
a). Inducible Defense Reactions
Inducible Defense Reactions are activated when a plant is wounded or attacked by a pathogen, initiating a series of protective responses. These include wound healing, where the plant forms a physical barrier by depositing materials like callose, lignin, and suberin at the cell walls to prevent further invasion, and morphological and cytological changes, such as the strengthening of cell walls through the accumulation of structural compounds to hinder pathogen penetration and the induction of programmed cell death (PCD or “Necrosis”), which involves the controlled death of infected cells to isolate and limit the spread of pathogens. These reactions collectively enhance the plant’s ability to defend against and recover from infections.
b). Hypersensitive Reaction
The hypersensitive reaction (HR) is a rapid and localized defense response in which plant cells at the site of infection undergo deliberate cell death to prevent pathogen spread, particularly effective against biotrophic pathogens that rely on living host tissue. The process begins when a pathogen, such as Phytophthora infestans, contacts a plant cell, triggering pathogen recognition through specific receptors.
This leads to cellular changes, including the reorganization of internal structures and the formation of granular material, culminating in necrotic cell death, where the infected cell dies in a controlled manner to isolate the pathogen and halt its progression.
Proteins Involved in Defense Reactions
Plants produce various defense proteins that play crucial roles in combating pathogens:
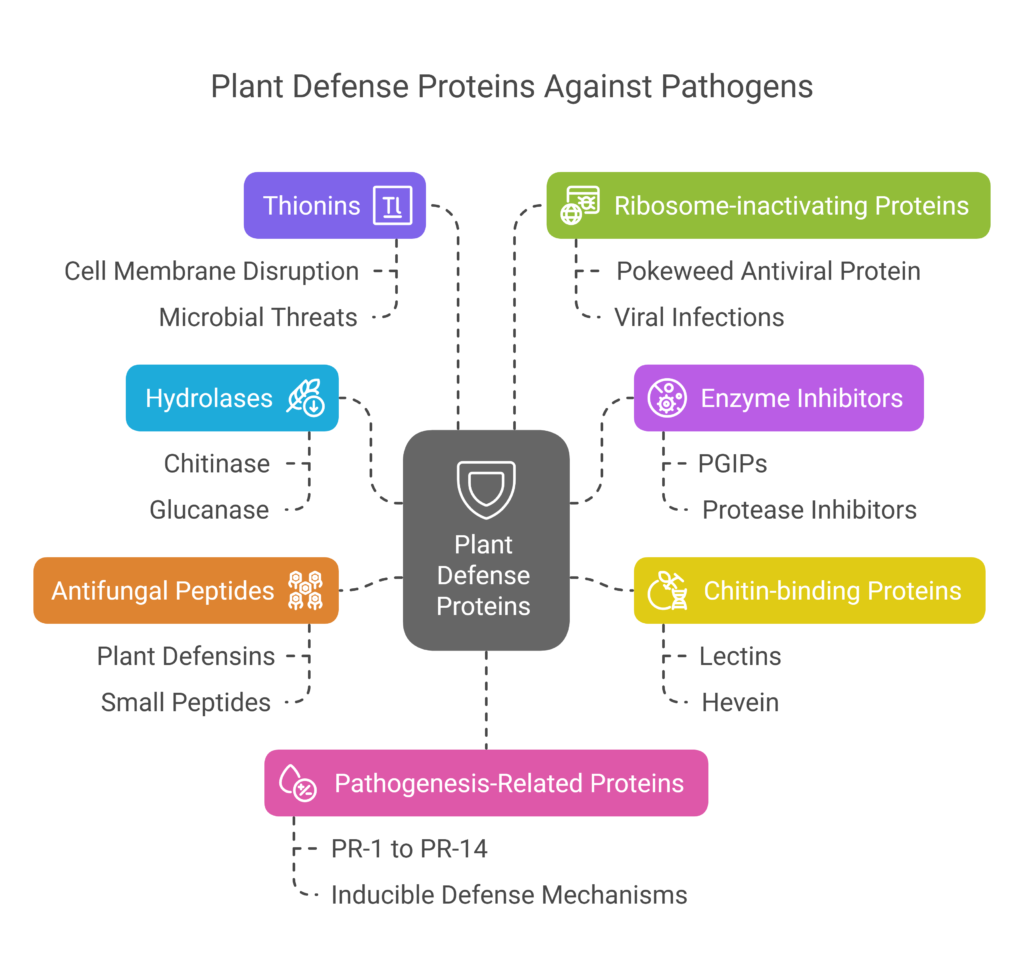
a). Hydrolases
Hydrolases are enzymes that play a critical role in plant defense by breaking down the structural components of fungal cell walls. Chitinase degrades chitin, a key polymer in fungal cell walls, while glucanase targets glucans, polysaccharides found in fungal and bacterial cell walls. By hydrolyzing these components, hydrolases weaken the structural integrity of pathogens, leading to cell wall disruption and pathogen death. These enzymes are often induced during pathogen attacks and work synergistically with other defense mechanisms to enhance the plant’s ability to combat infections effectively.
b). Enzyme Inhibitors
Enzyme inhibitors, such as polygalacturonase-inhibiting proteins (PGIPs), play a crucial role in plant defense by targeting and neutralizing pathogen enzymes. PGIPs specifically inhibit fungal pectinases, which are enzymes that break down plant cell walls, thereby preventing pathogen invasion. Other enzyme inhibitors, like protease and amylase inhibitors, target digestive enzymes in insects, disrupting their ability to feed and grow. These inhibitors are part of the plant’s strategy to block pathogen and pest activities at the molecular level.
c). Chitin-binding Proteins
A significant part of fungal cell walls and insect exoskeletons, chitin is bound by specialized proteins called chitin-binding proteins, which include lectins and hevein. These proteins impede the growth and development of insects and fungus by binding to chitin, hence preventing their capacity to infiltrate and harm plant tissues. This mechanism offers a focused and direct method of interfering with the life cycles of pests and pathogens.
d). Antifungal Peptides (AFPs)
Antifungal peptides (AFPs), such as plant defensins, are small, cysteine-rich peptides that exhibit strong antimicrobial activity. These peptides inhibit fungal growth by disrupting the integrity of fungal cell membranes or interfering with essential cellular processes. AFPs are often produced in response to pathogen attacks and provide a rapid and effective defense against fungal infections, contributing to the plant’s overall resistance.
e). Thionins
Thionins are small, cysteine-rich proteins that exhibit broad-spectrum antimicrobial activity. They inhibit the growth of both fungi and bacteria by disrupting the integrity of pathogen cell membranes or interfering with cellular functions. Thionins are often produced in response to pathogen attacks and play a key role in the plant’s innate immune system, providing a versatile defense against a wide range of microbial threats.
f). Ribosome-inactivating Proteins (RIPs)
Ribosome-inactivating proteins (RIPs), such as pokeweed antiviral protein (PAP), are specialized proteins that inhibit viral protein synthesis. RIPs achieve this by inactivating ribosomes, the cellular machinery responsible for protein production, thereby preventing viral replication. These proteins are particularly effective against viruses and play a crucial role in the plant’s defense against viral infections, helping to limit the spread and impact of viral pathogens.
g). Pathogenesis-Related (PR) Proteins
Pathogenesis-Related (PR) Proteins are a diverse group of proteins produced by plants in response to pathogen attacks, playing a crucial role in the plant’s immune system. These proteins, categorized as PR-1 to PR-14, perform a wide range of functions, including degrading pathogen structures like fungal cell walls, inhibiting pathogen enzymes such as pectinases, and producing antimicrobial compounds that directly target invading microbes. PR proteins are often induced during pathogen infection and contribute to the plant’s ability to resist and recover from diseases, making them a key component of the plant’s inducible defense mechanisms.
Phytoalexins for Enhancing Fungal Resistance in Plants
Phytoalexins are low molecular weight, antimicrobial compounds synthesized by plants in response to pathogen attacks or stress. Their small size enables rapid production and transport to infection sites, where they exhibit strong antimicrobial properties by inhibiting the growth of pathogens such as bacteria, fungi, and viruses. Unlike preformed defenses, phytoalexins are inducible, meaning they are specifically produced in reaction to pathogen invasion, making them a dynamic and targeted component of the plant’s immune response.
Role of Phytoalexins in Plant Defense
Phytoalexins play a vital role in plant defense by exhibiting direct antimicrobial activity, disrupting pathogen cellular functions such as membrane integrity and enzyme activity. They are produced in a localized response at the site of infection, creating a chemical barrier that limits pathogen spread. Additionally, phytoalexins work in synergistic effects with other defense mechanisms, such as the hypersensitive response and pathogenesis-related (PR) proteins, to enhance the plant’s overall resistance to pathogen attacks. This multifaceted approach ensures a robust and effective defense against invading pathogens.
Application of Small RNAs (sRNAs) and dsRNAs
One essential mechanism in plants for controlling gene expression and protecting against infections is the RNA interference (RNAi) pathway. A number of crucial steps in this pathway allow for exact regulation of gene activity. Double-stranded RNA (dsRNA) is first broken down into small interfering RNAs (siRNAs) by the enzyme Dicer.
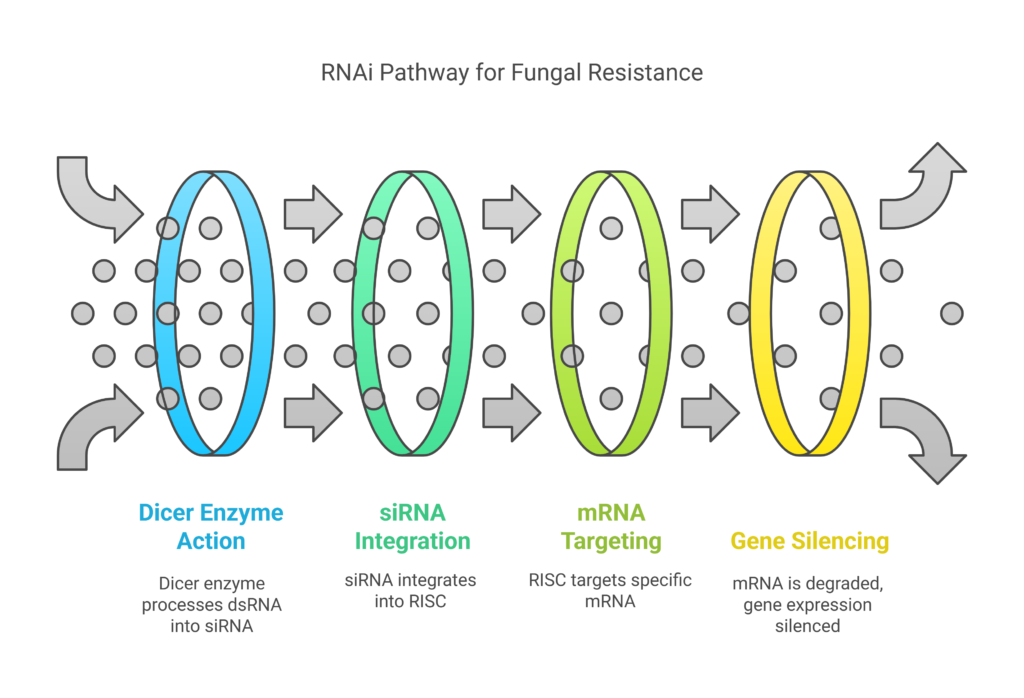
These siRNAs are subsequently integrated into the RNA-induced silencing complex (RISC), which locates and breaks down particular mRNA molecules using the siRNA as a guide. The process efficiently suppresses the expression of specific genes by breaking down these mRNAs, offering a potent defensive mechanism against infections.
A practical and innovative application of the RNAi pathway is Spray-Induced Gene Silencing (SIGS). This approach involves spraying plants with dsRNA designed to target specific genes in pathogens. When the pathogen takes up the dsRNA, the RNAi pathway is activated within the pathogen, leading to the silencing of essential genes and inhibiting its growth.
SIGS offers a targeted and environmentally friendly method for controlling fungal infections, reducing the need for chemical fungicides and enhancing plant resistance in a sustainable way.
Host-Induced Gene Silencing (HIGS)
A sophisticated technique called host-induced gene silencing (HIGS) allows plants to generate short RNAs (sRNAs) that are intended to target and quiet particular genes in pathogens. HIGS provides a targeted and sustainable method of disease control by altering key genes in the pathogen, increasing the plant’s resistance to a variety of challenges.
In the case of fungi, transgenic plants are engineered to express sRNAs that specifically target and silence crucial fungal genes. This disruption weakens the pathogen’s ability to infect and spread, providing the plant with robust resistance to fungal diseases.
For insect pests, plants can produce sRNAs that silence critical genes in the pests, such as those involved in growth, development, or survival. This reduces the viability of the pests, effectively limiting their ability to damage the plant and improving crop protection.
Similarly, against parasitic plants and nematodes, host plants generate sRNAs that travel through the phloem to reach and silence genes in these invaders. This process inhibits their ability to infect and harm the host plant, offering a natural and precise method of defense.
Overall, HIGS leverages the RNA interference (RNAi) pathway to provide a targeted, sustainable, and environmentally friendly solution for controlling plant diseases and pests. By harnessing the plant’s own genetic machinery, HIGS represents a significant advancement in agricultural biotechnology.
Genome Editing for Enhancing Fungal Resistance in Plants
CRISPR/Cas9 technology has revolutionized plant biology by enabling precise modifications to plant DNA, offering a powerful tool to enhance resistance to fungal pathogens. This genome-editing technique allows scientists to target and modify specific genes associated with susceptibility or resistance, providing a sustainable and efficient approach to crop protection.
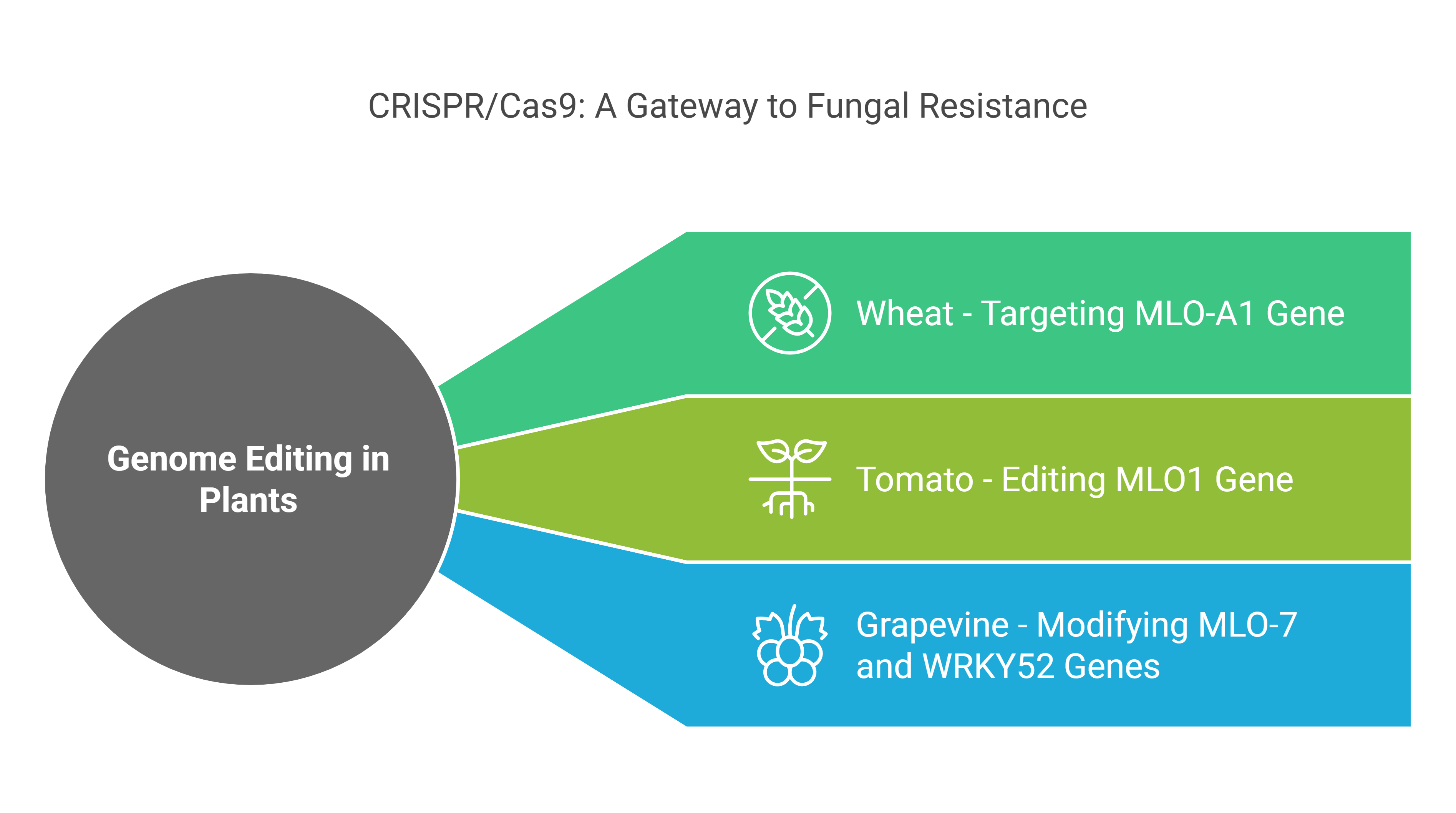
a). Wheat: Targeting the MLO-A1 Gene
In wheat, CRISPR/Cas9 has been used to target the MLO-A1 gene, which is associated with susceptibility to powdery mildew. By editing this gene, researchers have successfully conferred resistance to the disease, reducing the need for chemical fungicides and improving crop yields. This approach demonstrates the potential of CRISPR/Cas9 to develop disease-resistant wheat varieties.
b). Tomato: Editing the MLO1 Gene
Similarly, in tomatoes, the MLO1 gene has been edited using CRISPR/Cas9 to reduce vulnerability to powdery mildew. By disrupting this susceptibility gene, scientists have created tomato plants with enhanced resistance to the fungal pathogen, showcasing the versatility of genome editing in improving disease resistance across different crops.
c). Grapevine: Modifying the MLO-7 and WRKY52 Genes
In grapevines, CRISPR/Cas9 has been employed to modify genes such as MLO-7 and WRKY52, which are involved in resistance to powdery mildew and gray mold, respectively. These modifications enhance the plant’s ability to defend against fungal infections, highlighting the potential of genome editing to address multiple disease challenges in perennial crops.
Conclusion
Advancements in understanding plant defense mechanisms and the application of technologies like RNAi and CRISPR/Cas9 hold significant promises for developing crops with improved resistance to fungal pathogens. These strategies not only reduce reliance on chemical fungicides but also enhance agricultural sustainability.
Reference
Fister et al., 2018. Transient Expression of CRISPR/Cas9 Machinery Targeting TcNPR3 Enhances Defense Response in Theobroma cacao.
Nekrasov et al., 2017. Nekrasov, V., Wang, C., Win, J. et al. (2017). Rapid generation of a transgene-free powdery mildew resistant tomato by genome deletion.
Weiberg et al., 2015. Small RNAs — the secret agents in the plant–pathogen interactions.